|
Vol.
33 No. 6
November-December 2011
by Peter Atkins
As a contribution to the International Year of Chemistry, I decided to try to open the eyes of that elusive beast, the general public, to what lies at the core of chemistry: reactions. I decided to try to find a way to reveal the private life of atoms, what goes on at an atomic level, and what chemists hold in their mind’s eyes when they carry out a chemical reaction. I wanted to lead the reader from a vague knowledge that chemists bring about change to the hurly-burly life of atoms that the change represents. As I write in the preface to my recent book Reactions: The Private Life of Atoms:
. . . I have set out to help you understand and visualize the private lives of atoms so that when you look at chemical change—and chemical change is all around and within us, from the falling of a leaf through the digestion of food to the beating of a heart and even the forming of a thought, let alone the great industrial enterprises that manufacture the modern world—you will be able to imagine what is going on at a molecular scale. In the sections that follow, I invite you to imagine constructing a toolbox of fundamental processes which will enable you to imagine levering one atom away from its partner and encouraging it to join another. Then, with those basic tools in mind, I help you to establish a workshop where you will assemble the tools and bring them to bear on a variety of projects. Finally, I introduce you, in outline but not in detail, to how those workshops are invoked to engineer certain grand projects of construction.
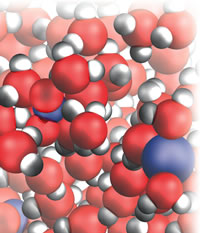 |
Figure 1: Silver nitrate solution. |
All of us are aware that the representation of atoms and molecules is fraught with danger and that the representation of the changes they undergo is even more hazardous. Nevertheless, I consider it essential to use visual images to convey what many of us might have in our mind’s eye when we think about chemistry, especially because images show that chemistry is all about tangible entities with characteristics that are the equivalent of personalities and which, like human personalities, lead them into a variety of combinations. The interplay of image and narrative was another challenge. I needed a relaxed, inviting tone, not that of an academic-textbook. I also wanted to achieve an effect in which the listening ear was able to glide with the watching eye into the images, so that the text was entwined with the illustrations, each reinforcing the role of the other.
I shall give some illustrations of the challenges and my solutions. So many of our reactions take place in the mobile world of water that I had to start there, and in particular with that simplest of all reactions, precipitation. After talking about water itself and the process of dissolving (of sodium chloride and silver nitrate, Fig 1), I write:
As soon as the solutions mix and the ions can mingle, the strong electrical attraction between the oppositely charged Ag+ and Cl– ions draws them together into little localized solid clumps, a powder. To us molecule-sized observers, the tiny particles of powder are like great rocks smashing down around us, thundering down from the solution overhead. The weak interactions between the Na+ ions and the smeared out charge of the NO3– ions are not strong enough to result in them clumping together: they remain in solution as a solution of soluble sodium nitrate. Precipitation reactions are about as simple as you can get in chemistry, the chemical equivalent of wife-swapping without the moral hesitation . . .
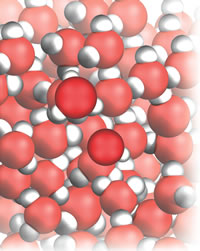 |
Figure 2: Water donating to itself. |
A step up in importance and interest lie acid-base reactions, Fig. 2, and here I develop the concept of proton transfer and the gradual widening of the conceptual net from Arrhenius to Brønsted (and later to Lewis):
When definitions are enlarged, like changing from fishing in coastal water to deep ocean, peculiar species are sometimes caught. Before we go on to see that the new definition captures everything that Arrhenius would regard as an acid (and then more), there is a very important, completely unexpected fish brought up in Lowry and Brønsted’s joint net. . . . Because two-faced water is not only an acid but also a base, then even before a conventional acid or base is added to a beaker of water, the molecules already present are both acids and bases. You now have to accept that when you drink a glass of water, you are drinking an acid. This is not a trivial conclusion to be shrugged off by saying that there somehow or other there probably isn’t much acid present. Every molecule is an acid, so you are drinking pure, highly concentrated acid. If you don’t like that thought, then you might like it even less to realize that you are also drinking a base. Once again, you can’t shrug off the thought by saying that the water is probably just a very dilute solution of a base. Every molecule is a base, so with every sip or gulp you are drinking highly concentrated, pure base. Such are the consequences of expanding and generalizing definitions: designed to catch sardines, they turn out to capture sharks.
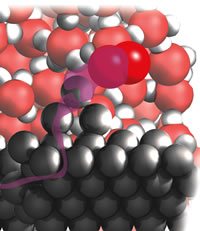 |
Figure 3: Reaction at the edge. |
One challenge that presented itself as soon as I reached redox reactions was how to convey the migration of an electron, as in the discussion of corrosion. I decided to use a waving transparent purple river to convey the sense of migration, Fig. 3:
I shall now show you the reaction in more detail and try to lead you into appreciating visually what is going on inside a small droplet of water on the surface of a sheet of rusting iron. Although rusting is rarely thought beautiful, there is a beauty and subtlety in the choreography of atomic events that underlie its formation. As usual, you should imagine shrinking to the size of a molecule, plunging below the droplet’s surface, and descending diver-like through the densely agitating, bustling, tumbling water molecules. You descend until you stand beside me on the hard surface of the virgin metal, among the rocky outcrops of iron atoms and the swirling eddies of water molecules.
From where we made metal-fall we strike out together towards the edge of the droplet, pushing through the water molecules. Near the edge we see oxygen molecules, O2, splashing in from the air beyond and wriggling into the depths between the water molecules. Then we see violent electronic action. When a sharklike O2 molecule reaches the iron surface it bites off two electrons from an Fe atom on an unwary outcrop. With two of its electrons gone, Fe has become Fe2+. It begins to fall away from the outcrop and starts to be surrounded by a clustering shell of H2O molecules . . .
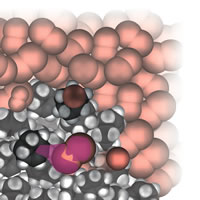 |
Figure 4: Starting addition. |
A perceptive reviewer of the original proposal worried that illustrations would all be dense collections of water molecules with the occasional glimpse of a reactant molecule. As it turned out, that was not the case, especially when I moved into the realm of organic reactions. Of course, there was another problem in this connection: which of the vast number of organic reactions should I choose? In the end I chose some typical addition and substitution reactions, Fig. 4. A further problem is that there is a lot going on during such reactions, and it is inevitable (I think) that the narrative gets more complicated:
Let’s shrink together down to our normal molecular size and watch what happens as the bromine is poured into the cyclohexene. We already know that a bromine molecule has a nose for negative charge, so you should not be surprised to see one homing in on the electron-rich double bond of a nearby cyclohexene molecule. We see a lot of rearrangement of the electron cloud once the bromine molecule is in contact with the double bond. In particular, some of that bond’s electron cloud bulges out from one C atom towards the exposed nucleus of the incoming molecule and starts to form a bond to it instead of to its original partner C atom. At the same time as that carbon-bromine bond starts to form, we see the original bromine-bromine bond starting to lengthen and weaken. At the end of this skirmish, a new carbon-bromine bond has formed, the old carbon-carbon double bond has dwindled to a single bond, the original bromine-bromine bond has broken, and the spare Br atom has drifted away as a Br- ion. Gone it might be, but it has duty to do shortly . . .
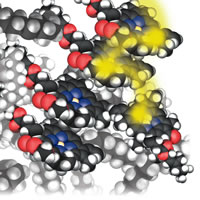 |
Figure 5: Energy dumping. |
Another “reactant” that needed thought is the photon. I needed to talk about photochemical processes, including photochemical smog and, more positively, vision and photosynthesis. I decided to depict a photon as a fuzzy yellow blob. At this stage in the presentation it was becoming impossible even to attempt to show in detail every step that was taking place in a complex biochemical process (the alternative was to write a whole other book). So, it became essential to focus on the core idea of what was taking place, Fig. 5:
The impacting photon stirs up the electron cloud on a chlorophyll molecule, which briefly becomes the home of the arriving energy. That energy is like a hot potato. We see it quickly tossed to a neighboring chlorophyll molecule, and then to another. In a twinkling of an eye (in more conventional units, in about one ten-billionth of a second) the energy jumps between about a thousand molecules. By passing on rapidly from molecule to molecule, the energy avoids degrading into molecular vibration (heat) or being emitted again as a photon, and is preserved for doing its job of synthesis. . . . The hot potato finally reaches the factory, the reaction center itself. It falls on to a pair of chlorophyll molecules and stirs up their electron clouds and then migrates to a closely related molecule, which is essentially a chlorophyll molecule without the central magnesium atom. The energy now is safely stored and can be used for construction by driving forward a string of proton transfer and redox reactions . . .
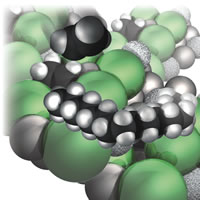 |
Figure 6: Polyethylene growing. |
I thought it important to bring the varieties of reactions together to show how combinations of them play crucial roles in the modern world. For instance, I wanted to give impressions of varieties of polymerization reactions, including the mechanism of Ziegler–Natta catalysis. Here, the challenge was not only to describe a fairly complex sequence of events, but also to depict what is happening at the surface of the solid catalyst, Fig. 6:
A much more controlled version of the polymerization minimizes the hair [the side chains and cross-linking of polyethylene]. As a result, the molecules are much more like ordinary spaghetti, bald spaghetti, and can pack together much more efficiently, giving a denser material. The key is a catalyst discovered by a German chemist, Karl Ziegler, and an Italian chemist, Giulio Natta, in the 1950s; it earned them the Nobel Prize in 1963 . . .
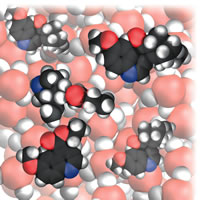 |
Figure 7: Joining the parts. |
More ambitiously, I wanted to give an impression—it could be no more than that—of how chemists deployed their awesome suite of tools to fabricate intricate architectural masterpieces, such as a quinine molecule, Fig. 7:
. . . You have arrived at your extraordinary destination, quinine. You are, perhaps, a little chagrined to realize that Cinchona Officinalis brainlessy achieves the same result in the quiet contemplation of its cells without all the paraphernalia you have needed to use and using a much more sophisticated step under the influence of enzymes. But that’s Nature. On the other hand, it is also quite remarkable that chemists have discovered how to emulate Her, and using the skills built up over a couple of hundred years to discover how to bend atoms to their will and build from them the equivalent of astonishing cathedrals.
Reactions: The Private Life of Atoms, by Peter Atkins, is published by Oxford University Press (2011).
Page
last modified 8 November 2011.
Copyright © 2003-2011 International Union of Pure and Applied Chemistry.
Questions regarding the website, please contact [email protected] |