|
Vol.
33 No. 2
March-April 2011
Where Would We Be
without Chemistry? by Peter Atkins
This year the world celebrates the International Year of Chemistry. The celebrations are wholly justified because chemistry is hugely important for all of us, wherever we live. Without chemistry’s contributions, the world would lack color; we would live in Stone Age conditions, underfed, dressed in skins, without the many devices that ease our lives and entertain us; and our lives would be short and painful. I will admit from the outset that chemistry, like any great enterprise, has a downside as well as an upside. It is used to make explosives for armaments, it creates poisons, and the effluents of chemistry plants can harm the environment. In the worst cases, chemical accidents have killed and injured thousands. The explosion at the Union Carbide plant in Bhopal, India, in 1984, for example, blighted thousands of innocent lives, and its terrible consequences are still with us today. While these negative aspects of chemistry have to be acknowledged, all technological and scientific advances have disadvantages, which we must weigh carefully against their advantages. With some exceptions, the chemical industry is well aware of its obligations to humanity and the environment and does what it can to avoid the potentially damaging effects of its activities.
This article will concentrate on chemistry’s positive contributions to the modern world and leave it to the reader to judge whether they outweigh its negatives.
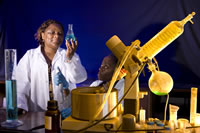 |
Professor Tebello Nyokong in her laboratory in the Department of Chemistry at Rhodes University of South Africa. One of five L’OREAL–UNESCO laureates in 2009, she earned the award for her research on chemical compounds known as phthalocyanine dyes. These could be used to attack cancerous tissues in a procedure that would be less intrusive than chemotherapy. |
Chemistry is the science of matter and the changes that matter can undergo. In the broadest possible terms, chemists take one form of matter and conjure from it a different form. In some cases, they take raw materials from the Earth, such as oil or ores, and produce other materials directly from them, such as petroleum fuels and iron for steel. Chemists might harvest the skies, taking nitrogen from the atmosphere and converting it to fertilizer. In many cases, they take more sophisticated forms of matter and convert them to materials suitable for use as fabrics or as substances needed for high technology. Communal Living Is Possible Thanks to Chemistry
Take water, for instance, the absolutely essential enabler of life. Chemistry has made communal living possible by purifying water and ridding it of pathogens. Chlorine is the principal agent in water purification: without chlorine, disease would be rampant, and urban living a gamble. Chemists have found ways of extracting this element from an abundant source: sodium chloride, or common salt. Yet is urban living possible without the environmental disadvantages of using chlorine? Can chemists (perhaps you the reader or one of your students whom you have encouraged to pursue the study of chemistry) find such a substitute for chlorine? A replacement for chlorine in this application is highly desirable because it is a dangerous and untrustworthy ally: although its potent chemical reactivity enables chlorine to purify water, that same reactivity enables chlorine to attack other compounds and enter the food chain as dioxins and related compounds. These compounds can attack the nervous system and accumulate in body fat. Chlorine and its compounds also rise high in the atmosphere where they contribute to the destruction of ozone and the formation of acid rain.
Chemists are at the forefront of the battle to obtain potable water from brackish water, from poisoned water in aquifers (such as the arsenic-laden water from deep aquifers in Bangladesh), and from that most abundant source of all, the oceans, by desalination. Chemists have contributed directly to this crucial task by developing reverse osmosis, a process in which pressure is applied to brackish water to drive it through a filtering membrane, thereby rendering it potable. Chemists have also contributed indirectly by developing membranes that promote the efficiency of the process by reducing its energy demands and increasing the lifetime and effectiveness of the membrane. It goes without saying that chemists’ traditional skills of analysis —discovering what is present in water, what can be tolerated, and what essential element should be removed to trigger the desired reaction—are vital to this endeavor. The Search for Explosives Spawns a Green Revolution
Then there is food. As the global population grows, and the productive land area on Earth is eroded, it becomes more and more important to coax crops into greater abundance. The traditional way to encourage abundance is to apply fertilizers. Here, chemists have made a huge contribution, in finding sources of nitrogen and phosphorus and ensuring that these can be assimilated by plants. Although controversial because of its possible interference with inheritable factors and the uncontrolled transfer of those factors into other species, genetic engineering is another exciting approach to increasing the food supply. Genetically modified (GM) food crops can reduce the need for chemical pesticides, resist viral infection and drought, and, like natural breeding, produce more abundant yields rich in desirable components. The introduction of golden rice, for example, which includes genes from yellow daffodils to provide a high concentration of precursors of vitamin A, might help millions of people in Africa and Southeast Asia who suffer from vitamin A deficiency, which is responsible for millions of deaths and thousands of cases of irreversible blindness.
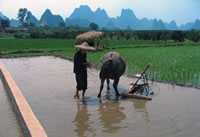 |
The introduction of golden rice, which includes genes from yellow daffodils, might help millions of people throughout Africa and Southeast Asia who suffer from vitamin A deficiency. |
Nitrogen is astonishingly abundant, making up nearly three-quarters of the atmosphere, but in its natural form it cannot be assimilated by most plants. One of chemistry’s greatest achievements, attained at the beginning of the 20th century under the impetus not of a humane desire to support life but of an inhumane desire to kill, was the discovery of a method for harvesting nitrogen from the air and turning it into a form that could be absorbed by crops. The original impetus of this discovery was the need to replace the natural source of nitrogen, nitrates mined in arid regions of Chile, since more abundant and reliable supplies of nitrogen were needed for the manufacture of explosives during World War I (1914–1918). The development in Germany of an effective, economical process for converting nonreactive gaseous nitrogen into a reactive form, by chemist Fritz Haber and his compatriot, the chemical engineer Carl Bosch, initially in 1909 and on an industrial scale in 1913, was a landmark achievement for the chemical industry for several reasons. As well as depending on the discovery of appropriate catalysts, it required the development of an industrial plant that operated at temperatures and pressures never previously attained.
The discovery of a process for producing reactive nitrogen revolutionized agriculture in the 20th century by permitting more abundant yields. But the process remains energy-intensive. It would be wonderful if the processes known to occur in certain bacteria associated with the root systems of leguminous plants, such as clover, alfalfa, and peanuts, could be emulated on an industrial scale to harvest nitrogen. In the natural process, nitrogen is released in a usable form when a plant dies and so becomes available to other plants. This is the basis for crop rotation in traditional farming and for the emulation of traditional methods in organic farming. Chemists have invested decades of research in investigating this natural process, dissecting in detail the enzymes that bacteria use in their quiet and energy-efficient, low-pressure, low-temperature way to produce usable nitrogen. There are glimmerings of success. If you want to go down in history as the chemist who cracked the problem of feeding the world, your opportunity may lie in continuing this important work.
Phosphorus—derived from the remains of prehistoric animals—is abundant, too. Calcium phosphate from the bones of these ancient animals, and molecules of adenosine triphosphate (ATP), which powered their cells, lie in great compressed heaps of phosphate rock below the world’s oceans and continents. Most of the world’s phosphate rock reserves (85 percent) are located in Morocco. Taken together, China and Morocco account for 91 percent of the world’s reserves, according to the International Fertilizer Development Center. Phosphorus is derived from phosphate rock and is used to produce fertilizers. By turning fossilized animals into fertilizer, chemists help to recycle the dead to feed the living.
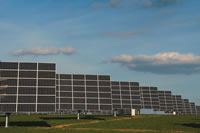 |
Without Energy, Civilizations Would Collapse
After water and food, we need energy. Nothing happens in the world without energy. Civilizations would collapse if it ceased to be available. Civilizations advance by deploying energy in ever greater abundance. Chemists contribute at all levels and to all aspects of developing both new sources of energy and more efficient applications of current sources.
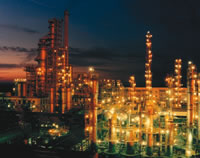 |
Petroleum is one of the legacies of the past, being the partially decomposed residue of organic matter, such as plankton and algae, that sank to the bottom of lakes and seas and was later subjected to heat and pressure. It is, of course, an extraordinarily convenient source of energy, as it can be transported easily, even in weight-sensitive aircraft. Chemists have long contributed to the refinement of this raw material, which is squeezed and pumped from the ground. They have developed processes and catalysts that have taken the molecules provided by nature, cut them into more volatile fragments, and reshaped them so that they burn more efficiently.
Of course, burning nature’s underground bounty might be seen, especially by future generations, as the wanton destruction of an invaluable resource. The supply of petroleum is also finite and, although new sources of petroleum are forever being discovered, for the time being at least, they are proving hazardous and increasingly expensive to access and use. Although an “empty” Earth is decades away, one day nonrenewable resources such as petroleum will be depleted. Chemists are already at work on the development of new sources of energy. Young people entering the field of chemistry today will find great opportunities to make an impact on the future well-being of the world and its people through the development of new energy sources.
Where do chemists currently look for new sources of energy? The Sun is an obvious source, and the capture of its energy adopted by nature, namely photosynthesis, is an obvious model to emulate. Chemists have already developed moderately efficient photovoltaic materials and continue to improve their efficiency. Nature, with its headstart of four billion years on laboratory chemists, has already developed a highly efficient system based on chlorophyll. Although the principal features of the process are understood, a challenge for current and future chemists is to adapt nature’s model to an industrial scale. One route is to use sunlight to split water (H2O) into its component elements and to pipe or pump the hydrogen to where it can be burned.
I say “burned,” but chemists know that there are more subtle and efficient ways of using the energy that hydrogen and hydrocarbons represent than igniting them, capturing the energy released as heat, and using that heat in a mechanical, inefficient engine or electrical generator. Electrochemistry, the use of chemical reactions to generate electricity and the use of electricity to bring about chemical change, is potentially of huge importance to the world. Chemists have already helped to produce the mobile sources, the batteries, that drive our small portable appliances, such as lamps, music players, laptops, telephones, and monitoring devices of all kinds, as well as, increasingly, our cars.
In collaboration with engineers, chemists are deeply involved in the development of fuel cells on all scales, from driving laptops to powering entire homes and conceivably villages. In a fuel cell, electricity is generated by allowing chemical reactions to dump and extract electrons into and from conducting surfaces while fuel, either hydrogen or hydrocarbons, is supplied from outside. The viability of a fuel cell depends crucially on the nature of the surfaces where the reactions take place and the medium in which the cells are immersed. This is another area of chemistry in which the aspiring chemist could make a profound difference in the future of his or her country and the world.
Even nuclear power, both fission and one day fusion, the emulation on Earth of the Sun, depends on the skills of chemists. The construction of reactors for nuclear fission depends on the availability of new materials. The extraction of nuclear fuel in the form of uranium and oxides from its ores involves chemistry. Holding back the development and public acceptance of nuclear energy, apart from political and economic concerns, is the problem of how to dispose of the spent radioactive fuel. Chemists are contributing to solutions to this problem by identifying ways to extract useful isotopes from nuclear waste and to ensure that it does not enter the environment and become a hazard for centuries to come. Chemists who collaborate with nuclear engineers to solve the problem of nuclear waste will help both to reduce the risks of nuclear power and to facilitate the development of less hazardous nuclear fusion. Nuclear fusion involves smashing isotopes of hydrogen together and capturing the energy released as they merge to form helium, as happens on the Sun. The challenge in nuclear fusion is to achieve high temperatures, because only then do the nuclei smash together with sufficient force to overcome their electrical repulsion—and to avoid melting the entire apparatus. The major nuclear fusion research effort in France, known as the International Thermonuclear Experimental Reactor (ITER) project (iter is Latin for “the way”), is an international project on an unprecedented scale, involving countries representing half the world’s population. Plastics from Oil
I have alluded to the seemingly wanton destruction of an invaluable resource when the complex organic mixture we know as oil is sucked from the ground where it has lain for millennia, then casually burned. Of course, not all the oil goes through the exhausts of our cars, trucks, trains, and aircraft, Much is extracted and used more productively in an awesome chain of reactions that chemists have developed which constitute the petrochemicals industry.
Of the many petrochemical products, plastics have probably made the greatest impact on our lives. A century ago, everyday objects were metallic, ceramic, or natural, that is, made from such materials as wood, wool, cotton, and silk. Today, many objects that we use on a daily basis are synthetics derived from oil. Our clothes and fabrics in our homes are synthetics spun from materials developed by chemists; we travel with bags and cases formed from synthetics; our electronic equipment—televisions, telephones, music players, and laptops—are molded from synthetics. Our vehicles are increasingly fabricated from synthetics. The look and feel of the world is different today from what it was a hundred years ago: touch an object today, and its texture will typically be synthetic. For this transformation, we are indebted to chemists.
Even if you lament the passing of many natural materials, you can still thank chemists for their preservation when they are used. Natural matter rots, but chemists have developed materials that can slow decay. New wood preservatives, for instance, have been developed to avoid problems associated with old preservatives, which were typically based on copper and could leach into the soil, poisoning it with arsenic, copper, and chromium. Lighter Cars, Molecular Computers, and Intelligent Clothing
Plastics are but one face of the materials revolution that has characterized the last hundred years and continues vigorously today. Chemists are also developing ceramics that are beginning to replace the metals used in vehicles, making them lighter and thereby increasing the efficiency of our transport systems. Ceramics are already used in the exhaust manifolds of some high-performance cars, and experiments have been conducted to replace the entire engine block with ceramics. Ceramics are a factor in the simplification of the car engine’s cooling system and help today’s engines withstand high temperatures. Problems with fabrication and crack resistance still await chemists’ solutions.
Chemists are also responsible for the development of the semiconductors that form the material infrastructure of the digital world and enable communications and computation. Chemists have been involved in developing optical fibers to replace copper in the transmission of signals. Materials developed by chemists make possible the displays that act as interfaces with the human visual system.
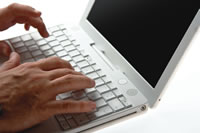 |
Currently, chemists are at work on molecular computers, in which switches and memory are based on changes in the shape of molecules. The successful development of such materials will result in an unprecedented increase in computational power and an astonishing compactness of the processing unit. If you are interested in the development of such smart materials, you can expect to participate in a revolution in computation. Quantum computing is another exciting prospect, which will depend on appropriate new materials developed by chemists and represent an extraordinary revolution in communication and computation.
Modern fabrics depend crucially on chemistry. Take chemistry away from clothing and fashion, and we are left almost naked, cold, and most certainly drab. Traditional dyes, such as those used in Javanese batik and Indian block-printing, are chemicals that have been extracted from plants for application to fabric. Modern fabrics include synthetics such as polyesters, nylon, and polyamides. Chemistry also makes more subtle contributions to fabrics: it incorporates bromine compounds into fabrics to act as flame retardants, and nanomaterials to prolong resistance to chafing, create resistance to bacteria, and suppress wrinkling. Even more exciting developments to which you might contribute are on the horizon: e-textiles (so-called intelligent clothing) with embedded electronic capabilities, including the ability to change colors and patterns (and even display advertisements!) that reflect our moods. Such textiles will be able to adjust their thermal properties to the ambient conditions and, let’s hope, be self-cleaning. Agents against Disease: Pharmaceutical Companies
I cannot neglect to mention the role of chemistry in health care. One of chemistry’s greatest contributions to our well-being—and, it must be added, to the welfare of domesticated animals—has been the development of pharmaceuticals. Chemists can be justly proud of their contributions to the development of a variety of agents against pain and disease. The introduction of anesthetics in the late 19th and early 20th centuries was an especially welcome contribution. Think of undergoing an amputation 200 years ago, with only brandy and gritted teeth to sustain you! Some of the anesthetics currently used, such as procaine, have been developed specifically to avoid adverse side effects, including addiction, which often accompanied the use of such traditional materials as cocaine, derived from Peruvian coca. Chemists have also developed a vast array of antibiotics, often by observing nature closely. A century ago, bacterial infection was a deadly prospect; now it is treatable with antibiotics. We hope that it remains that way, but we still need to prepare for the unknown or unforeseen.
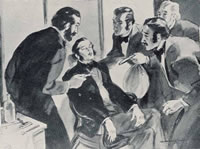 |
This 19th-century patient has been given nitrous oxide (N20) to numb the pain of having a tooth extracted. Also known as laughing gas, nitrous oxide was identified as an anesthetic in 1772 by the English
chemist Joseph Priestley. |
Pharmaceutical companies often come under attack for what many regard as profligate profits and exploitation. Yet their underlying motive is admirable: to reduce human suffering by developing drugs that conquer pain and combat disease. Chemists are at the heart of this endeavor. It is highly regrettable that drug development can be so expensive. Modern computational techniques have been useful in the search for new lines of approach and in the effort to reduce reliance on animal testing. Extraordinary care needs to be exercised when introducing foreign materials into living bodies, and years of costly research can suddenly be trashed if unacceptable consequences are discovered at the final stage of testing. Chemists’ involvement in the pharmaceutical industry might transform it in a manner we cannot yet foresee. You or one of your colleagues may one day become one of the proud chemists who have contributed to saving millions of lives. How Biology Became Chemistry
Closely allied with chemists’ contribution to the alleviation of disease is their involvement in research at a molecular level. Biology became chemistry just over 50 years ago when the double helix structure of DNA was discovered. Molecular biology, which sprung in large measure from that discovery, is chemistry applied to organisms. Chemists, often in the guise of molecular biologists, have opened the door to understanding life and its principal characteristic, inheritance, at a most fundamental level through the rational investigation of vast areas of the molecular world. Their work has also transformed both forensic medicine and anthropology, helping to bring criminals to justice and to trace ethnic origins and ancestry.
Chemistry’s shift of attention to the processes of life has occurred at a time when the traditional branches of chemistry—organic, inorganic, and physical—have reached a stage of considerable maturity and are ready to focus on the extremely complex network of processes going on inside organisms—inside human bodies in particular. Discoveries by chemists have helped create a rational basis for approaches to the treatment and prevention of disease. Genomics and proteomics are of crucial importance to chemists who work in this field. As its name suggests, genomics is the study of the genomes of organisms. So far, the human genome and those of several other animal species have been mapped. The term “proteomics,” which combines the words “protein” and “genomics,” refers to the study of the proteins produced by an organism. In these areas of chemistry you can truly feel confident about standing on the shoulders of the giants who have preceded you, and know that you are attacking disease at its roots. Magicians of Matter
I have focused on a few of the achievements of applied chemistry, for they are the tangible outcome of the labors of myriad working chemists over the ages, as well as, it must be said with some caution, the alchemists, who were, above all, motivated by the desire to turn base metals into gold.
There is, however, another aspect of chemistry that should not go unnoticed and which, for many, is the real justification for the pursuit of chemistry. Chemistry provides insight into matter and the workings of the material world. It is thus a deeply cultural pursuit. It is fitting then that, in the light of UNESCO’s support of the International Year of Chemistry, chemistry should be at once educational, scientific, and cultural. Chemistry not only opens our inner eye to the properties and behavior of matter. It is also a truly transnational and transcultural activity, in which advances build on contributions from chemists in almost every country in the world.
The early chemists like Englishman John Dalton (1766–1844) brought the existence of atoms and molecules to our attention, and their successors have shown us how to relate those entities to what we observe. Although we can take pleasure from merely looking at the vibrant color of a flower, it is through chemistry that we can perceive the molecular origin of the color and thus deepen our delight.
Muhammad ibn Zakariya ar-Razi (865–925) was a Persian alchemist, chemist, physician, and philosopher. Many “firsts” are attributed to him, including being the first to differentiate smallpox from measles and to write a book on pediatrics. He was also the first to discover sulfuric acid, after perfecting the methods of distillation and extraction. He discovered numerous other chemicals and compounds, including kerosene, alcohol, and ethanol.
The early chemists began to understand why one substance reacted with another but not with something else. Those who followed in their footsteps discovered the motive power of chemical change and thereby expanded our understanding of why things happen in the world. We understand what drives the world forward, why crops grow, why we live and die, and why anything happens at all.
Much, of course, remains to be done. Although the fundamental principles of chemistry are now well established, their application remains as challenging and vigorously pursued as ever. Chemistry lets us plumb the depths of matter, and fabricate subtle arrangements of atoms that might not exist anywhere else in the universe, and that possess properties that are exquisitely tuned for a hitherto unforeseen application. If you are, or intend to become, a chemist, you will become a magician of matter, able to conjure unexpected or intended new forms of matter from what surrounds us. Of course, you will not be an actual magician: rather, you will be a rational, understanding manipulator, an architect on the scale of molecules.
The International Year of Chemistry is rightly a celebration of the transformation of the world and the lives of its inhabitants by chemistry. It rightly celebrates current achievements of chemists, the impact of chemistry on people everywhere, and the advancement of the field by collaboration throughout the world. It also rightly anticipates chemistry’s important contributions to the new world yet to be. Peter Atkins is recently retired as a professor of chemistry at Oxford University and fellow of Lincoln College. He has written “the” textbooks on chemistry, including Physical Chemistry (9 editions!), Inorganic Chemistry, Physical Chemistry for the Life Sciences, and Molecular Quantum Mechanics. He is also the author of several more popular books including The Periodic Kingdom, Atkins’ Molecules, Galileo’s Finger, and Four Laws That Drive the Universe. His latest book On Being—A Scientist’s Exploration of the Great Questions of Existence is to be published this year, as will Reactions—The Private Life of Atoms.
This feature was jointly
published in A World of Science, the UNESCO Natural Science Quarterly Newsletter (January 2011).
Page
last modified 8 March 2011.
Copyright © 2003-2011 International Union of Pure and Applied Chemistry.
Questions regarding the website, please contact [email protected] |