Chemistry International
Vol. 21, No. 6
November 1999
News
from IUPAC
Biodegradation
of Chemical Warfare Agents
Newer CW Biodegradation Research Efforts
Show Progress
Several Approaches to Biodegrading Nerve Agents
Other Microorganisms with CW Hydrolytic Enzymes Identified
Strategies for Degrading Bulk Agents
Biodegrading the Blistering Agents HD and HT
Strategies for Degrading Organo-arsenical
Blistering Agents
Unanswered CW Degradation Questions
Require Further Research
Acknowledgments
Suggested Reading
This article by Dr. Walter Mulbry ([email protected]),
a microbiologist at the Soil Microbial Systems Laboratory, USDA/ARS,
Beltsville, MD, USA, and Evgenia Rainina, a microbiologist at the Department
of Biochemistry and Biophysics, Texas A&M University, College Station,
TX, USA, constitutes the report of a Working Party of the IUPAC Ad Hoc
Committee on Chemical Weapons Destruction Technologies (chaired by Professor
Joseph F. Bunnett, [email protected]).
It was originally published in ASM News, Vol. 64, No. 6, 1998,
pp. 325-331, and it is reprinted here with the kind permission
of the American Society for Microbiology. We thank the authors and Patrick
Lacey ([email protected]),
Production Editor at ASM News, for making it possible for us
to publish this report.
The Chemical Weapons Convention (CWC), which the U.S.
government ratified in 1997, sets an explicit timetable for signatory
countries to destroy their chemical weapon stockpiles and related facilities.
Although destroying such agents and remediating contaminated sites by
conventional means promise to be enormously costly processes, recent
research indicates that some of these compounds can be biologically
degraded, suggesting an environmentally and economically feasible alternative
strategy for addressing these challenges.
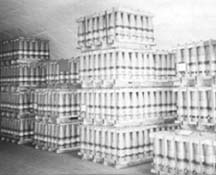
Figure 1. Artillery shells containing the nerve
gas sarin (GB) inside a storage bunker at the Umatilla Chemical
Depot in Oregon. (Photo courtesy of Donna Fuzi, Department of
the Army)
|
The magnitude of the U.S. chemical weapon (CW) stockpileover
30 000 tons of various blister and nerve agentspresents a
formidable challenge to those charged with disposing of it. About 60%
of the agents are stored in steel 1-ton bulk containers; the remaining
40% are loaded in several million explosively configured rockets, land
mines, mortars, bombs, artillery projectiles, and spray tanks (Figure
1). The "youngest" CW munitions and storage tanks are 30 years
old, and the oldest are 53. In addition, U.S. Army officials have identified
215 sites in 33 states that are likely to contain buried chemical weapons
or to be contaminated with chemical agents.
The financial resources needed for these tasks are equally
formidable. Since 1985, the U.S. Army has spent USD 3.2 billion on its
programs for destroying the U.S. CW stockpile and on planning for the
treatment of material at nonstockpile sites (about 4% of these funds
were used for research and development). The current cost estimate for
the stockpile disposal program, USD 12.4 billion, has increased sevenfold
since 1985 and is likely to increase further. In addition, U.S. Army
officials estimate that at least another USD 16.6 billion will be needed
over the next 40 years to treat buried material at nonstockpile sites.
Although few of the other CWC signatory nations have major
CW stockpiles to deal with, several of the independent republics of
the former Soviet Union, whose financial resources are already strapped,
face cleanup tasks comparable to that of the United States. Other known
CW concentrations include Japanese CW munitions abandoned in China in
1945 and an estimated 100 000 tons of German CW munitions that were
dumped into the Baltic Sea at the end of World War II.
Newer CW Biodegradation Research
Efforts Show Progress
For more than 50 years, the U.S. military and its counterparts in other
countries have disposed of obsolete and surplus chemical weapons by
a variety of means that are no longer acceptable. For instance, prior
to 1969, the U.S. Army disposed of chemical weapons by open-pit burning,
evaporative "atmospheric dilution," burial, and placement
of munitions in concrete coffins for ocean dumping. In the 1970s, the
U.S. Army began using alkaline hydrolysis to deactivate mustard agents
known as H and HD (Figure 2).
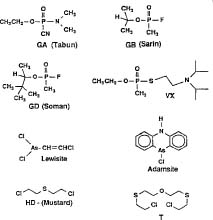
Figure 2. Chemical structures of the primary
chemical warfare agents present in the U.S. and Russian stockpiles
|
Because of problems with GB alkaline hydrolysis, U.S. Army officials
in 1982 adopted incineration for destroying all classes of chemical
weapons. Subsequently, a full-scale CW incinerator facility was built
in the Marshall Islands on Johnson Atoll and, more recently, a similar
incinerator was built and brought into operation in Utah. Strong and
effective opposition to incineration has, however, stalled U.S. Army
plans to operate incinerators at seven other U.S. CW stockpiles. Similarly,
strong opposition has stalled CW incineration projects in the independent
republics of the former Soviet Union.
Historically, chemists and engineers who were involved
in developing CW agents also were the specialists who studied how to
dispose of these agents. However, in the late 1980s, the U.S. Army began
a small intramural program on biodegradation at their Edgewood Research,
Development and Engineering Center (ERDEC) in Aberdeen, MD. From 1991-1996,
this program was broadened to support extramural research at several
universities, including Texas A&M University, Rutgers University,
and the University of Washington. In Russia, civilian research groups
at Moscow State University, the Pushchino Biological Research Center,
and several other government institutes have also been working on disposal
strategies for agents in their stockpile.
These biodegradative research efforts are showing progress,
particularly with efforts to dispose safely of both nerve and blister
agents. Indeed, faced with strong political pressure to avoid incinerating
such agents, U.S. Army officials recently changed CW destruction plans
at one stockpile site, formally assigning biodegradation a role in treating
HD stored in bulk containers.
Table 1. Bacterial strains with hydrolytic activities
against CW nerve agents
Several Approaches to Biodegrading
Nerve Agents
The organophosphorus (OP) "nerve gases" include
VX, GA (tabun), GB (sarin), and GD (soman), all of which are liquid
with varied volatility at room temperature (Figure 2). The blistering
agents are oily liquids at room temperature and include the "mustard"
agents H, HD (agent H that has been purified by distillation), and HT
(a 60/40 mixture of HD and agent T), as well as the organo-arsenical
agent lewisite (L). Adamsite (DA), a second organo-arsenical agent,
is a severe respiratory irritant. Mixtures of the mustard and arsenical
agents were also produced, such as agent MLM (mustard-lewisite
mixture, a 37/63 mixture of agents HD and L).
In the 1980s, investigators began to search for microorganisms
that could metabolize CW agents or structurally related compounds. In
the search for microorganisms to act on nerve agents, researchers focused
on microbial isolates capable of hydrolyzing OP insecticides. Among
a range of microbially derived OP insecticide hydrolyzing enzymes, only
the enzyme OPH from Pseudomonas diminuta is at all active in
degrading nerve agents (Table 1).
Recently, James Wild and his colleagues at Texas A&M
University modified the cloned gene for OPH, changing the enzyme's catalytic
specificity and increasing its ability to degrade two organophosphonate
nerve agents. Thus, following site-directed mutagenesis, cells displayed
a 4-fold increase in activity against VX and a 40-fold increase in activity
against soman.
Collaborating researchers in other laboratories at Texas
A&M University, the U.S. Army ERDEC, the University of Wisconsin,
and the University of Pittsburgh are helping to define the catalytic
and structural capabilities of the native and modified forms of OPH.
For example, in 1994, Hazel Holden and her colleagues at the University
of Wisconsin determined a crystal structure for this protein, which
can be downloaded from the Brookhaven Protein Data Base on the World
Wide Web at http://pdb.pdb.bnl.gov/ (PDB code 1PTA).
Other Microorganisms with CW Hydrolytic
Enzymes Identified
In 1989, reasoning that microbial enzymes used in decontamination
may need to be resistant to high salt concentrations, Joe DeFrank at
the U.S. Army's ERDEC screened isolates from a hypersaline spring in
Utah for CW agent hydrolytic activities. Among those isolates, he and
his colleagues found several related Alteromonas isolates that
efficiently hydrolyze nerve agents such as GB, GD, and GF (Table 1).
Subsequently, DeFrank and his colleagues Steve Harvey
and Tu-chen Cheng at ERDEC characterized two related enzymes from these
Alteromonas strains and isolated the gene (opaA) that
encodes one of these enzymes (termed OPAA-2). The DNA sequence of opaA
shares a 28% amino acid homology to Escherichia coli aminopeptidase
P and human prolidase. Moreover, OPAA-2 is highly active in hydrolyzing
two dipeptide substrates of prolidase, namely Leu-Pro and Ala-Pro, but
is not active against tripeptide substrates of aminopeptidase P or the
dipeptides Pro-Leu or Pro-Gly. Such evidence suggests that OPAA-2 is
a prolidase having a role in peptide metabolism.
Although no further screening efforts have been undertaken,
a more comprehensive screening of microbial dipeptidase activities from
different sources may yield entirely new enzymes with higher activities
or activities toward other nerve agents such as GA and VX.
Strategies for Degrading Bulk
Agents
The Chemical Weapons Convention specifies not only that
CW agents be destroyed irreversibly but also that particular by-products
be destroyed to ensure that they cannot be reused for military purposes.
The by-products also need to be rendered safe for discharge into open
environments. Hence, the members of several research groups are studying
whether biodegradation will serve to destroy the by-products remaining
following either chemical or enzymatic hydrolysis of CW agents. This
research has yielded promising results for treating both nerve and mustard
agents.
For example, Robin Autenrieth and her collaborators from
Texas A&M University and the U.S. Army's ERDEC are studying the
steps required to generate an environmentally acceptable waste stream
following hydrolysis of sarin. She proposes hydrolyzing sarin with an
excess of sodium hydroxide, a process that yields a solution containing
sodium isopropyl methylphosphonate
(IMPA) and sodium fluoride, both of which must be processed before
discharge (Figure 3).
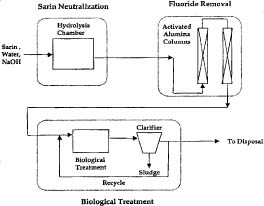
Figure 3. Schematic of a proposed strategy
for the treatment of sarin from bulk containers. In this process,
sarin is chemically hydrolyzed using excess sodium hydroxide,
fluoride is removed by absorption to alumina columns, and the
resulting effluent is treated in bioreactors. (Figure courtesy
of Robin Autenrieth, Texas A&M University)
|
Although attempts to use IMPA as a carbon source to support
microbial growth were not successful, IMPA is metabolized as a sole
source of phosphorus in bioreactors. In batch experiments where sarin
hydrolysate is supplied to microorganisms growing in bioreactors, IMPA
levels decrease from 85 mg/liter to their detection limit of 1 mg/liter
within 60-75 hours, depending on the microbial consortia in use.
However, the consortia require a six-week acclimation period, are inhibited
by high levels of the sarin hydrolysate or other sources of phosphate,
and require significant amounts of carbon and nitrogen to achieve the
required C:N:P ratio of 100:12:1.
The structurally similar nerve agent soman can be treated
by comparable hydrolytic and biodegradation steps. However, to reduce
the acclimation periods and to raise growth rates when suspended cultures
are supplied with soman hydrolysate, Evgenia Rainina at Texas A&M
University tested whether immobilized microbial cells metabolize propylmethylphosphonic
acid (PMPA) (the primary product of soman hydrolysis) as a sole phosphorus
source.
For such experiments, a PMPA-degrading strain from the
ERDEC collection is first grown to a high density in rich medium, immobilized
within a poly(vinyl) alcohol cryogel, and incubated in phosphate-limited
media for 24 hours to reduce endogenous phosphorus pools. In successive
batch experiments using immobilized cells, PMPA levels decrease from
164 mg/liter to the detection limit of 1 mg/liter within 60 hours. These
results demonstrate the potential utility of the cell immobilization
technique for maintaining high enzymatic activities.
Applied separately, water or hydrogen peroxide can effectively
hydrolyze another organophosphonate agent, VX, providing a promising
first step along the path to degrading this compound. Whether water
or hydrogen peroxide is used, ethylmethylphosphonic acid (EMPA) is a
by-product that needs to be further degraded. It, too, can serve as
a sole phosphorus source for natural microbial consortia in bioreactors.
However, the use of VX, soman, or sarin hydrolysates as
phosphorus sources requires the addition of excess nitrogen and carbon,
and is rate-limiting. Introducing cells containing constitutive C-P
lyase activities into these consortia might accelerate the overall degradative
process and thereby reduce the need for adding large amounts of nitrogen
and carbon.
Biodegrading the Blistering
Agents HD and HT
The poorly soluble blistering agent HD reacts with microbial
proteins and is therefore highly toxic to microbial cells, making it
a poor candidate for direct biodegradation. Alkaline hydrolysis of HD
yields significant amounts of polymerized by-products that also are
difficult to biodegrade, according to Yu-Chu Yang and colleagues at
the U.S. Army's ERDEC. However, Steve Harvey and his colleagues at ERDEC
recently determined that water at 90 oC effectively hydrolyzes
HD, yielding 90-95% thiodiglycol (TDG), which is nontoxic and miscible
in water.
Because the CWC mandates destruction of TDG, Harvey has
been testing an aerobic sequencing batch reactor for its ability to
biodegrade hydrolyzed HD products. Using activated municipal sludge
as an inoculum and HD hydrolysate as a sole carbon source, this batch
process yields a nontoxic effluent. Meanwhile, a cryoimmobilized culture
of Alcaligenes xylosoxidans from ERDEC metabolizes 150 mM TDG
within 24 hours and retains 100% of its initial activity after 4 months
of continuous use, according to Evgenia Rainina.
Although the two-step strategy of hydrolysis followed
by biodegradation readily applies to bulk containers of HD (purified
mustard) and HT, its applicability to stockpiled U.S. munitions containing
HD or agent H (unpurified mustard) is less certain. The stockpiles of
other nations include the less expensive agent H rather than agent HD.
Depending on how those materials were manufactured, agent H typically
contains about 30% impurities and may also contain an array of thickeners
and other additives. In many cases, during the decades of storage these
materials have reacted with one another and with metal casing materials,
forming solid or semisolid polymers that are recalcitrant to degradation.
(NEXT)